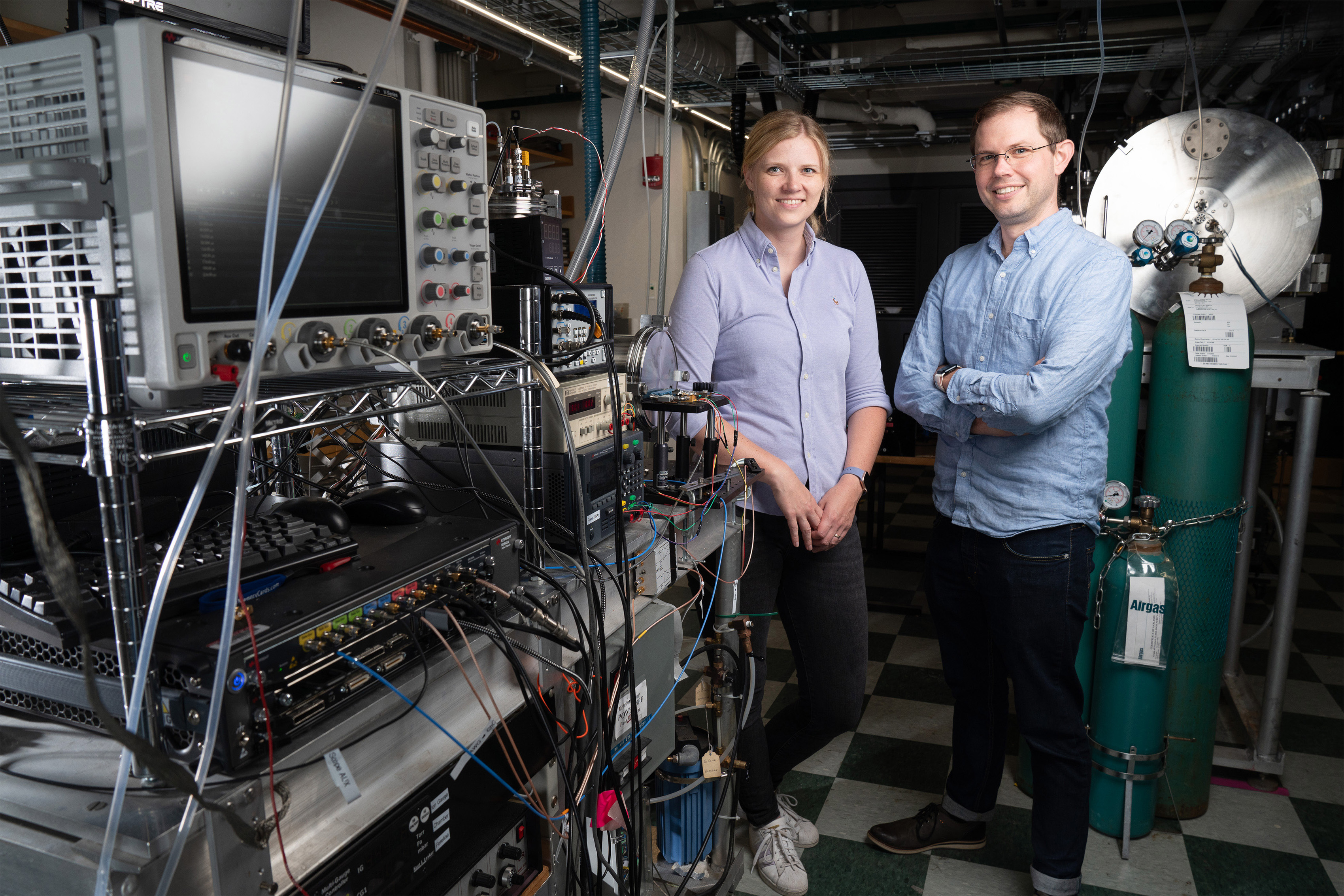
A team led by researchers at MIT has discovered that a distant interstellar cloud contains an abundance of pyrene, a type of large, carbon-containing molecule known as a polycyclic aromatic hydrocarbon (PAH).
The discovery of pyrene in this far-off cloud, which is similar to the collection of dust and gas that eventually became our own solar system, suggests that pyrene may have been the source of much of the carbon in our solar system. That hypothesis is also supported by a recent finding that samples returned from the near-Earth asteroid Ryugu contain large quantities of pyrene.
“One of the big questions in star and planet formation is: How much of the chemical inventory from that early molecular cloud is inherited and forms the base components of the solar system? What we’re looking at is the start and the end, and they’re showing the same thing. That’s pretty strong evidence that this material from the early molecular cloud finds its way into the ice, dust, and rocky bodies that make up our solar system,” says Brett McGuire, an assistant professor of chemistry at MIT.
Due to its symmetry, pyrene itself is invisible to the radio astronomy techniques that have been used to detect about 95 percent of molecules in space. Instead, the researchers detected an isomer of cyanopyrene, a version of pyrene that has reacted with cyanide to break its symmetry. The molecule was detected in a distant cloud known as TMC-1, using the 100-meter Green Bank Telescope (GBT), a radio telescope at the Green Bank Observatory in West Virginia.
McGuire and Ilsa Cooke, an assistant professor of chemistry at the University of British Colombia, are the senior authors of a paper describing the findings, which appears today in Science. Gabi Wenzel, an MIT postdoc in McGuire’s group, is the lead author of the study.
Carbon in space
PAHs, which contain rings of carbon atoms fused together, are believed to store 10 to 25 percent of the carbon that exists in space. More than 40 years ago, scientists using infrared telescopes began detecting features that are thought to belong to vibrational modes of PAHs in space, but this technique couldn’t reveal exactly which types of PAHs were out there.
“Since the PAH hypothesis was developed in the 1980s, many people have accepted that PAHs are in space, and they have been found in meteorites, comets, and asteroid samples, but we can’t really use infrared spectroscopy to unambiguously identify individual PAHs in space,” Wenzel says.
In 2018, a team led by McGuire reported the discovery of benzonitrile — a six-carbon ring attached to a nitrile (carbon-nitrogen) group — in TMC-1. To make this discovery, they used the GBT, which can detect molecules in space by their rotational spectra — distinctive patterns of light that molecules give off as they tumble through space. In 2021, his team detected the first individual PAHs in space: two isomers of cyanonaphthalene, which consists of two rings fused together, with a nitrile group attached to one ring.
On Earth, PAHs commonly occur as byproducts of burning fossil fuels, and they’re also found in char marks on grilled food. Their discovery in TMC-1, which is only about 10 kelvins, suggested that it may also be possible for them to form at very low temperatures.
The fact that PAHs have also been found in meteorites, asteroids, and comets has led many scientists to hypothesize that PAHs are the source of much of the carbon that formed our own solar system. In 2023, researchers in Japan found large quantities of pyrene in samples returned from the asteroid Ryugu during the Hayabusa2 mission, along with smaller PAHs including naphthalene.
That discovery motivated McGuire and his colleagues to look for pyrene in TMC-1. Pyrene, which contains four rings, is larger than any of the other PAHs that have been detected in space. In fact, it’s the third-largest molecule identified in space, and the largest ever detected using radio astronomy.
Before looking for these molecules in space, the researchers first had to synthesize cyanopyrene in the laboratory. The cyano or nitrile group is necessary for the molecule to emit a signal that a radio telescope can detect. The synthesis was performed by MIT postdoc Shuo Zhang in the group of Alison Wendlandt, an MIT associate professor of chemistry.
Then, the researchers analyzed the signals that the molecules emit in the laboratory, which are exactly the same as the signals that they emit in space.
Using the GBT, the researchers found these signatures throughout TMC-1. They also found that cyanopyrene accounts for about 0.1 percent of all the carbon found in the cloud, which sounds small but is significant when one considers the thousands of different types of carbon-containing molecules that exist in space, McGuire says.
“While 0.1 percent doesn’t sound like a large number, most carbon is trapped in carbon monoxide (CO), the second-most abundant molecule in the universe besides molecular hydrogen. If we set CO aside, one in every few hundred or so remaining carbon atoms is in pyrene. Imagine the thousands of different molecules that are out there, nearly all of them with many different carbon atoms in them, and one in a few hundred is in pyrene,” he says. “That is an absolutely massive abundance. An almost unbelievable sink of carbon. It’s an interstellar island of stability.”
Ewine van Dishoeck, a professor of molecular astrophysics at Leiden Observatory in the Netherlands, called the discovery “unexpected and exciting.”
“It builds on their earlier discoveries of smaller aromatic molecules, but to make the jump now to the pyrene family is huge. Not only does it demonstrate that a significant fraction of carbon is locked up in these molecules, but it also points to different formation routes of aromatics than have been considered so far,” says van Dishoeck, who was not involved in the research.
An abundance of pyrene
Interstellar clouds like TMC-1 may eventually give rise to stars, as clumps of dust and gas coalesce into larger bodies and begin to heat up. Planets, asteroids, and comets arise from some of the gas and dust that surround young stars. Scientists can’t look back in time at the interstellar cloud that gave rise to our own solar system, but the discovery of pyrene in TMC-1, along with the presence of large amounts of pyrene in the asteroid Ryugu, suggests that pyrene may have been the source of much of the carbon in our own solar system.
“We now have, I would venture to say, the strongest evidence ever of this direct molecular inheritance from the cold cloud all the way through to the actual rocks in the solar system,” McGuire says.
The researchers now plan to look for even larger PAH molecules in TMC-1. They also hope to investigate the question of whether the pyrene found in TMC-1 was formed within the cold cloud or whether it arrived from elsewhere in the universe, possibly from the high-energy combustion processes that surround dying stars.
The research was funded in part by a Beckman Foundation Young Investigator Award, the Schmidt Family Futures Foundation, the U.S. National Science Foundation, the Natural Sciences and Engineering Research Council of Canada, the Goddard Center for Astrobiology, and the NASA Planetary Science Division Internal Scientist Funding Program.