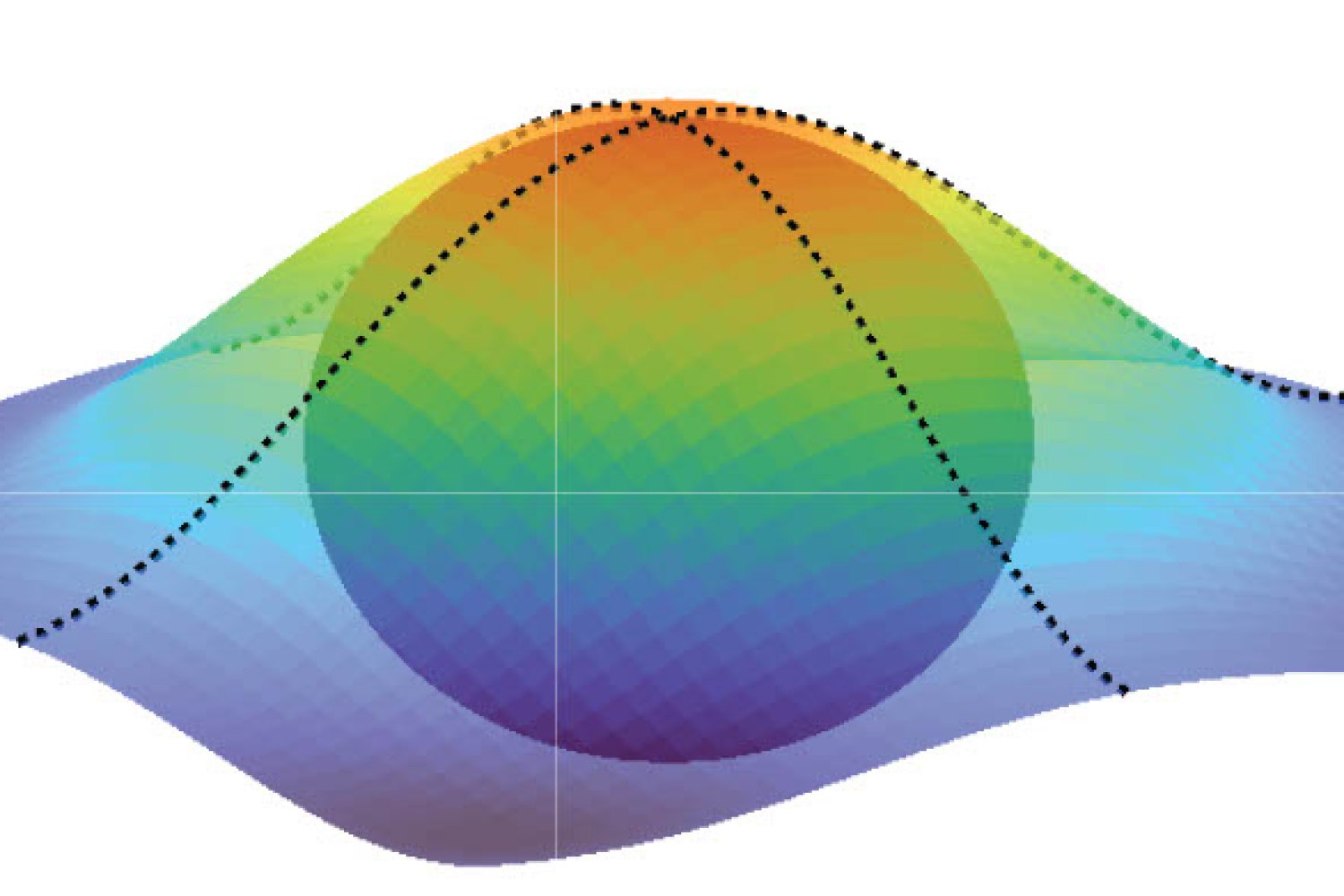
MIT physicists and colleagues have for the first time measured the geometry, or shape, of electrons in solids at the quantum level. Scientists have long known how to measure the energies and velocities of electrons in crystalline materials, but until now, those systems’ quantum geometry could only be inferred theoretically, or sometimes not at all.
The work, reported in the Nov. 25 issue of Nature Physics, “opens new avenues for understanding and manipulating the quantum properties of materials,” says Riccardo Comin, MIT’s Class of 1947 Career Development Associate Professor of Physics and leader of the work.
“We’ve essentially developed a blueprint for obtaining some completely new information that couldn’t be obtained before,” says Comin, who is also affiliated with MIT’s Materials Research Laboratory and the Research Laboratory of Electronics.
The work could be applied to “any kind of quantum material, not just the one we worked with,” says Mingu Kang PhD ’23, first author of the Nature Physics paper who conducted the work as an MIT graduate student and who is now a Kavli Postdoctoral Fellow at Cornell University’s Laboratory of Atomic and Solid State Physics.
Kang was also invited to write an accompanying research briefing on the work, including its implications, for the Nov. 25 issue of Nature Physics.
A weird world
In the weird world of quantum physics, an electron can be described as both a point in space and a wave-like shape. At the heart of the current work is a fundamental object known as a wave function that describes the latter. “You can think of it like a surface in a three-dimensional space,” says Comin.
There are different types of wave functions, ranging from the simple to the complex. Think of a ball. That is analogous to a simple, or trivial, wave function. Now picture a Mobius strip, the kind of structure explored by M.C. Escher in his art. That’s analogous to a complex, or nontrivial, wave function. And the quantum world is filled with materials composed of the latter.
But until now, the quantum geometry of wave functions could only be inferred theoretically, or sometimes not at all. And the property is becoming more and more important as physicists find more and more quantum materials with potential applications in everything from quantum computers to advanced electronic and magnetic devices.
The MIT team solved the problem using a technique called angle-resolved photoemission spectroscopy, or ARPES. Comin, Kang, and some of the same colleagues had used the technique in other research. For example, in 2022 they reported discovering the “secret sauce” behind exotic properties of a new quantum material known as a kagome metal. That work, too, appeared in Nature Physics. In the current work, the team adapted ARPES to measure the quantum geometry of a kagome metal.
Close collaborations
Kang stresses that the new ability to measure the quantum geometry of materials “comes from the close cooperation between theorists and experimentalists.”
The Covid-19 pandemic, too, had an impact. Kang, who is from South Korea, was based in that country during the pandemic. “That facilitated a collaboration with theorists in South Korea,” says Kang, an experimentalist.
The pandemic also led to an unusual opportunity for Comin. He traveled to Italy to help run the ARPES experiments at the Italian Light Source Elettra, a national laboratory. The lab was closed during the pandemic, but was starting to reopen when Comin arrived. He found himself alone, however, when Kang tested positive for Covid and couldn’t join him. So he inadvertently ran the experiments himself with the support of local scientists. “As a professor, I lead projects, but students and postdocs actually carry out the work. So this is basically the last study where I actually contributed to the experiments themselves,” he says with a smile.
In addition to Kang and Comin, additional authors of the Nature Physics paper are Sunje Kim of Seoul National University (Kim is a co-first author with Kang); Paul M. Neves, a graduate student in the MIT Department of Physics; Linda Ye of Stanford University; Junseo Jung of Seoul National University; Denny Puntel of the University of Trieste; Federico Mazzola of Consiglio Nazionale delle Ricerche and Ca’ Foscari University of Venice; Shiang Fang of Google DeepMind; Chris Jozwiak, Aaron Bostwick, and Eli Rotenberg of Lawrence Berkeley National Laboratory; Jun Fuji and Ivana Vobornik of Consiglio Nazionale delle Ricerche; Jae-Hoon Park of Max Planck POSTECH/Korea Research Initiative and Pohang University of Science and Technology; Joseph G. Checkelsky, associate professor of physics at MIT; and Bohm-Jung Yang of Seoul National University, who co-led the research project with Comin.
This work was funded by the U.S. Air Force Office of Scientific Research, the U.S. National Science Foundation, the Gordon and Betty Moore Foundation, the National Research Foundation of Korea, the Samsung Science and Technology Foundation, the U.S. Army Research Office, the U.S. Department of Energy Office of Science, the Heising-Simons Physics Research Fellow Program, the Tsinghua Education Foundation, the NFFA-MUR Italy Progetti Internazionali facility, the Samsung Foundation of Culture, and the Kavli Institute at Cornell.