AI is becoming a more significant part of our lives every day. But as powerful as it is, many AI systems still work like “black boxes.” They make decisions and predictions, but it’s hard to understand how they reach those conclusions. This can make people hesitant…
OmniOps Secures $8 Million to Accelerate Saudi Arabia’s AI Transformation
OmniOps, a Saudi Arabia-based AI infrastructure technology provider founded in 2024 by entrepreneur Mohammed Altassan, has secured SAR 30 million (approximately $8 million) in funding from GMS Capital Ventures. This anchor investment will fuel OmniOps’ mission to optimize and scale AI workloads while driving sustainable, energy-efficient…
AI governance: Analysing emerging global regulations
Governments are scrambling to establish regulations to govern AI, citing numerous concerns over data privacy, bias, safety, and more. AI News caught up with Nerijus Šveistys, Senior Legal Counsel at Oxylabs, to understand the state of play when it comes to AI regulation and its potential…
AI and data analytics-driven finance transformation
AI and data analytics are valuable assets for discovering real-time insights, proactive decision-making, and predictive capabilities….
What might happen if AI can feel emotions? – AI News
In a world where artificial intelligence is becoming omnipresent, it’s fascinating to think about the prospect of AI-powered robots and digital avatars that can experience emotions, similar to humans. AI models lack consciousness and they don’t have the capacity to feel emotions, but what possibilities might…
The BEST Spoon Graphics Tutorials from 2024
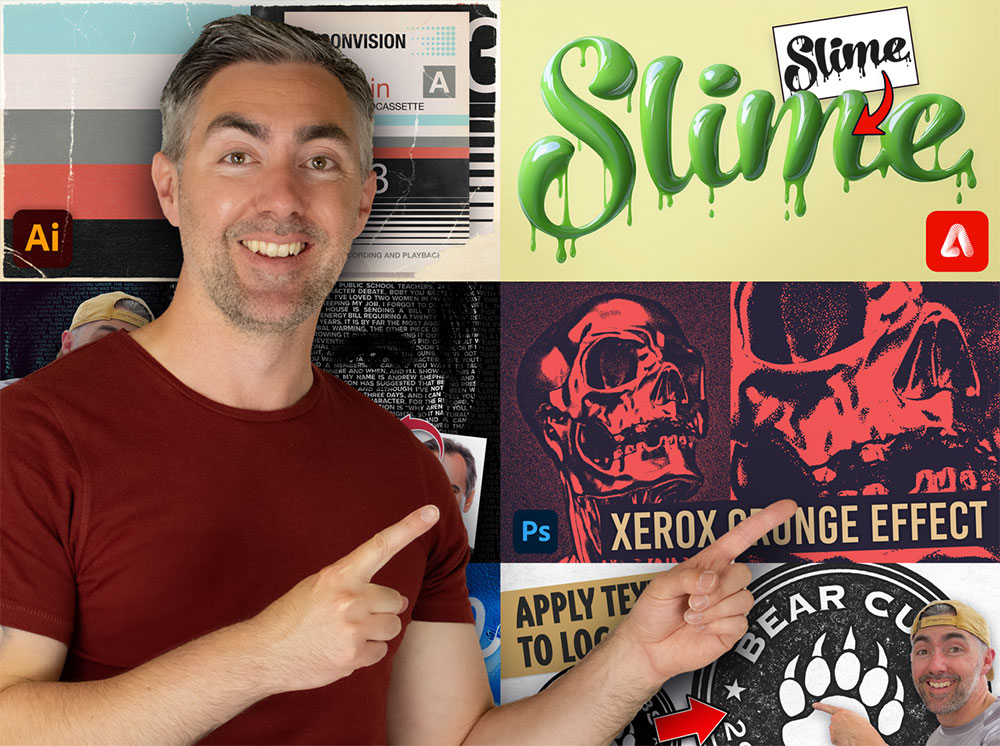
2024 is coming to an end, so let’s take a look back at my tutorial videos from the past year and see which topics proved to be the most popular. Once again my Neon Light Effect Photoshop Tutorial from back in 2021 continues to dominate my YouTube Analytics as my best performing video, now with 2.8 million total views. Sadly, none of my uploads this year have managed to pull out any extraordinary numbers, my best performing videos are just about on par with 9th and 10th place from my 2023 roundup! I thought my recent Vaporwave Neon Effect Photoshop Tutorial might have followed in the footsteps of that popular Neon Light Effect tutorial with it being the same process with a different result, but the stats aren’t quite living up to expectations yet with 3000 views so far. Hopefully this will be one to watch if the algorithm decides to shine some light on it in 2025.
► Neon Light Effect Photoshop Tutorial – https://youtu.be/3JxCbwn-6bQ
► Vaporwave Neon Effect Photoshop Tutorial – https://youtu.be/Ht21wUDPS-M
► Easily Create Fire in Photoshop With This Incredible Flames Action – https://youtu.be/oT2CyMCbQXM
► EASILY Create NEON Effects With AI in Adobe Firefly – https://youtu.be/NF3XORW2iMA
► Adobe Illustrator Tutorial: Design a Retro VHS Cover Inspired Poster – https://youtu.be/Br6XI0TwYyA
► 10 Font Styles Every Graphic Designer Should Know – https://youtu.be/GowuIcIabKs
► Add Textures in Photoshop with the Hidden “KNOCKOUT” Method – https://youtu.be/Ewyn-yoNFoc
► How to Create Watercolour Painting Effects with AI Using Photoshop’s Generative Fill – https://youtu.be/XmNFlgCGWpw
► Easy Green Slime Text Effect With Adobe Firefly – https://youtu.be/0bm3SRvSa_k
► Photoshop Pencil Sketch Effect Tutorial – https://youtu.be/DWQMNKSw2XA
► Text Portrait Effect in Photoshop – https://youtu.be/gF2qujOMtVI
► Grainy Xerox Grunge Effect Photoshop Tutorial – https://youtu.be/fnTdmhODwW8
AI Breakthroughs in Endoscopy
Artificial intelligence (AI) has vast potential in the medical field. It’s particularly valuable in procedures like endoscopy that, despite being common, require complex analysis and expert insight. The health care industry hasn’t overlooked this opportunity, either, as early uses of AI in endoscopy have already driven…
10 Best AI Tools for Musicians (December 2024)
Artificial intelligence is not just augmenting today’s music production – it is fundamentally reimagining how musicians create, practice, and interact with sound. From advanced stem separation to natural language synthesis, these tools represent the cutting edge of what is possible when neural networks meet musical creativity….
The Hidden Role of Data Annotation in Everyday AI Tools
A recent survey of 6,000 consumers revealed something intriguing: while only around 33% of people think they use AI, a remarkable 77% are, in fact, using AI-powered services or devices in their daily lives. This gap highlights how many people may not realize how much artificial…