He develops new high-performance energy storage systems for solar and wind power: Prof. Yulong Ding from the University…
Say Hello to Biodegradable Microplastics – Technology Org
Microplastics are tiny, nearly indestructible fragments shed from everyday plastic products. As we learn more about microplastics, the…
Aiming exascale at black holes – Technology Org
In 1783, John Michell, a rector in northern England, “proposed that the mass of a star could reach…
Signs of life detectable in single ice grain emitted from extraterrestrial moons – Technology Org
The ice-encrusted oceans of some of the moons orbiting Saturn and Jupiter are leading candidates in the search…
Large language models use a surprisingly simple mechanism to retrieve some stored knowledge
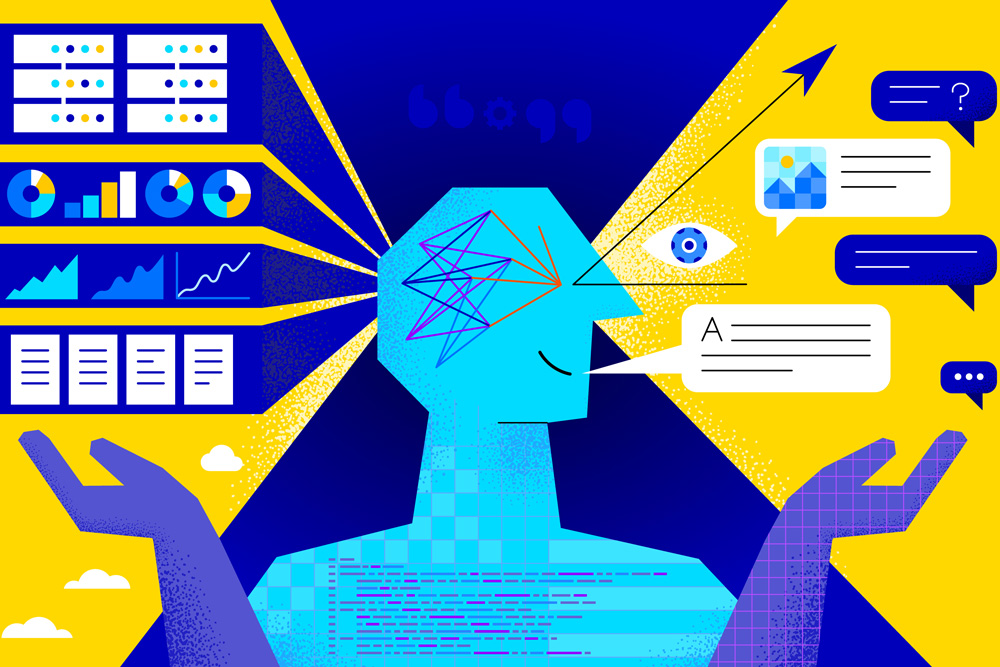
Large language models, such as those that power popular artificial intelligence chatbots like ChatGPT, are incredibly complex. Even though these models are being used as tools in many areas, such as customer support, code generation, and language translation, scientists still don’t fully grasp how they work.
In an effort to better understand what is going on under the hood, researchers at MIT and elsewhere studied the mechanisms at work when these enormous machine-learning models retrieve stored knowledge.
They found a surprising result: Large language models (LLMs) often use a very simple linear function to recover and decode stored facts. Moreover, the model uses the same decoding function for similar types of facts. Linear functions, equations with only two variables and no exponents, capture the straightforward, straight-line relationship between two variables.
The researchers showed that, by identifying linear functions for different facts, they can probe the model to see what it knows about new subjects, and where within the model that knowledge is stored.
Using a technique they developed to estimate these simple functions, the researchers found that even when a model answers a prompt incorrectly, it has often stored the correct information. In the future, scientists could use such an approach to find and correct falsehoods inside the model, which could reduce a model’s tendency to sometimes give incorrect or nonsensical answers.
“Even though these models are really complicated, nonlinear functions that are trained on lots of data and are very hard to understand, there are sometimes really simple mechanisms working inside them. This is one instance of that,” says Evan Hernandez, an electrical engineering and computer science (EECS) graduate student and co-lead author of a paper detailing these findings.
Hernandez wrote the paper with co-lead author Arnab Sharma, a computer science graduate student at Northeastern University; his advisor, Jacob Andreas, an associate professor in EECS and a member of the Computer Science and Artificial Intelligence Laboratory (CSAIL); senior author David Bau, an assistant professor of computer science at Northeastern; and others at MIT, Harvard University, and the Israeli Institute of Technology. The research will be presented at the International Conference on Learning Representations.
Finding facts
Most large language models, also called transformer models, are neural networks. Loosely based on the human brain, neural networks contain billions of interconnected nodes, or neurons, that are grouped into many layers, and which encode and process data.
Much of the knowledge stored in a transformer can be represented as relations that connect subjects and objects. For instance, “Miles Davis plays the trumpet” is a relation that connects the subject, Miles Davis, to the object, trumpet.
As a transformer gains more knowledge, it stores additional facts about a certain subject across multiple layers. If a user asks about that subject, the model must decode the most relevant fact to respond to the query.
If someone prompts a transformer by saying “Miles Davis plays the. . .” the model should respond with “trumpet” and not “Illinois” (the state where Miles Davis was born).
“Somewhere in the network’s computation, there has to be a mechanism that goes and looks for the fact that Miles Davis plays the trumpet, and then pulls that information out and helps generate the next word. We wanted to understand what that mechanism was,” Hernandez says.
The researchers set up a series of experiments to probe LLMs, and found that, even though they are extremely complex, the models decode relational information using a simple linear function. Each function is specific to the type of fact being retrieved.
For example, the transformer would use one decoding function any time it wants to output the instrument a person plays and a different function each time it wants to output the state where a person was born.
The researchers developed a method to estimate these simple functions, and then computed functions for 47 different relations, such as “capital city of a country” and “lead singer of a band.”
While there could be an infinite number of possible relations, the researchers chose to study this specific subset because they are representative of the kinds of facts that can be written in this way.
They tested each function by changing the subject to see if it could recover the correct object information. For instance, the function for “capital city of a country” should retrieve Oslo if the subject is Norway and London if the subject is England.
Functions retrieved the correct information more than 60 percent of the time, showing that some information in a transformer is encoded and retrieved in this way.
“But not everything is linearly encoded. For some facts, even though the model knows them and will predict text that is consistent with these facts, we can’t find linear functions for them. This suggests that the model is doing something more intricate to store that information,” he says.
Visualizing a model’s knowledge
They also used the functions to determine what a model believes is true about different subjects.
In one experiment, they started with the prompt “Bill Bradley was a” and used the decoding functions for “plays sports” and “attended university” to see if the model knows that Sen. Bradley was a basketball player who attended Princeton.
“We can show that, even though the model may choose to focus on different information when it produces text, it does encode all that information,” Hernandez says.
They used this probing technique to produce what they call an “attribute lens,” a grid that visualizes where specific information about a particular relation is stored within the transformer’s many layers.
Attribute lenses can be generated automatically, providing a streamlined method to help researchers understand more about a model. This visualization tool could enable scientists and engineers to correct stored knowledge and help prevent an AI chatbot from giving false information.
In the future, Hernandez and his collaborators want to better understand what happens in cases where facts are not stored linearly. They would also like to run experiments with larger models, as well as study the precision of linear decoding functions.
“This is an exciting work that reveals a missing piece in our understanding of how large language models recall factual knowledge during inference. Previous work showed that LLMs build information-rich representations of given subjects, from which specific attributes are being extracted during inference. This work shows that the complex nonlinear computation of LLMs for attribute extraction can be well-approximated with a simple linear function,” says Mor Geva Pipek, an assistant professor in the School of Computer Science at Tel Aviv University, who was not involved with this work.
This research was supported, in part, by Open Philanthropy, the Israeli Science Foundation, and an Azrieli Foundation Early Career Faculty Fellowship.
Engineering household robots to have a little common sense
From wiping up spills to serving up food, robots are being taught to carry out increasingly complicated household tasks. Many such home-bot trainees are learning through imitation; they are programmed to copy the motions that a human physically guides them through.
It turns out that robots are excellent mimics. But unless engineers also program them to adjust to every possible bump and nudge, robots don’t necessarily know how to handle these situations, short of starting their task from the top.
Now MIT engineers are aiming to give robots a bit of common sense when faced with situations that push them off their trained path. They’ve developed a method that connects robot motion data with the “common sense knowledge” of large language models, or LLMs.
Their approach enables a robot to logically parse many given household task into subtasks, and to physically adjust to disruptions within a subtask so that the robot can move on without having to go back and start a task from scratch — and without engineers having to explicitly program fixes for every possible failure along the way.
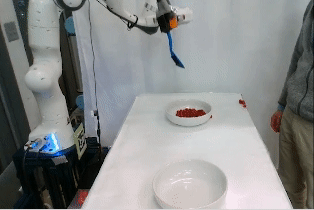
“Imitation learning is a mainstream approach enabling household robots. But if a robot is blindly mimicking a human’s motion trajectories, tiny errors can accumulate and eventually derail the rest of the execution,” says Yanwei Wang, a graduate student in MIT’s Department of Electrical Engineering and Computer Science (EECS). “With our method, a robot can self-correct execution errors and improve overall task success.”
Wang and his colleagues detail their new approach in a study they will present at the International Conference on Learning Representations (ICLR) in May. The study’s co-authors include EECS graduate students Tsun-Hsuan Wang and Jiayuan Mao, Michael Hagenow, a postdoc in MIT’s Department of Aeronautics and Astronautics (AeroAstro), and Julie Shah, the H.N. Slater Professor in Aeronautics and Astronautics at MIT.
Language task
The researchers illustrate their new approach with a simple chore: scooping marbles from one bowl and pouring them into another. To accomplish this task, engineers would typically move a robot through the motions of scooping and pouring — all in one fluid trajectory. They might do this multiple times, to give the robot a number of human demonstrations to mimic.
“But the human demonstration is one long, continuous trajectory,” Wang says.
The team realized that, while a human might demonstrate a single task in one go, that task depends on a sequence of subtasks, or trajectories. For instance, the robot has to first reach into a bowl before it can scoop, and it must scoop up marbles before moving to the empty bowl, and so forth. If a robot is pushed or nudged to make a mistake during any of these subtasks, its only recourse is to stop and start from the beginning, unless engineers were to explicitly label each subtask and program or collect new demonstrations for the robot to recover from the said failure, to enable a robot to self-correct in the moment.
“That level of planning is very tedious,” Wang says.
Instead, he and his colleagues found some of this work could be done automatically by LLMs. These deep learning models process immense libraries of text, which they use to establish connections between words, sentences, and paragraphs. Through these connections, an LLM can then generate new sentences based on what it has learned about the kind of word that is likely to follow the last.
For their part, the researchers found that in addition to sentences and paragraphs, an LLM can be prompted to produce a logical list of subtasks that would be involved in a given task. For instance, if queried to list the actions involved in scooping marbles from one bowl into another, an LLM might produce a sequence of verbs such as “reach,” “scoop,” “transport,” and “pour.”
“LLMs have a way to tell you how to do each step of a task, in natural language. A human’s continuous demonstration is the embodiment of those steps, in physical space,” Wang says. “And we wanted to connect the two, so that a robot would automatically know what stage it is in a task, and be able to replan and recover on its own.”
Mapping marbles
For their new approach, the team developed an algorithm to automatically connect an LLM’s natural language label for a particular subtask with a robot’s position in physical space or an image that encodes the robot state. Mapping a robot’s physical coordinates, or an image of the robot state, to a natural language label is known as “grounding.” The team’s new algorithm is designed to learn a grounding “classifier,” meaning that it learns to automatically identify what semantic subtask a robot is in — for example, “reach” versus “scoop” — given its physical coordinates or an image view.
“The grounding classifier facilitates this dialogue between what the robot is doing in the physical space and what the LLM knows about the subtasks, and the constraints you have to pay attention to within each subtask,” Wang explains.
The team demonstrated the approach in experiments with a robotic arm that they trained on a marble-scooping task. Experimenters trained the robot by physically guiding it through the task of first reaching into a bowl, scooping up marbles, transporting them over an empty bowl, and pouring them in. After a few demonstrations, the team then used a pretrained LLM and asked the model to list the steps involved in scooping marbles from one bowl to another. The researchers then used their new algorithm to connect the LLM’s defined subtasks with the robot’s motion trajectory data. The algorithm automatically learned to map the robot’s physical coordinates in the trajectories and the corresponding image view to a given subtask.
The team then let the robot carry out the scooping task on its own, using the newly learned grounding classifiers. As the robot moved through the steps of the task, the experimenters pushed and nudged the bot off its path, and knocked marbles off its spoon at various points. Rather than stop and start from the beginning again, or continue blindly with no marbles on its spoon, the bot was able to self-correct, and completed each subtask before moving on to the next. (For instance, it would make sure that it successfully scooped marbles before transporting them to the empty bowl.)
“With our method, when the robot is making mistakes, we don’t need to ask humans to program or give extra demonstrations of how to recover from failures,” Wang says. “That’s super exciting because there’s a huge effort now toward training household robots with data collected on teleoperation systems. Our algorithm can now convert that training data into robust robot behavior that can do complex tasks, despite external perturbations.”
New Neural Model Enables AI-to-AI Linguistic Communication
In a significant leap forward for artificial intelligence (AI), a team from the University of Geneva (UNIGE) has successfully developed a model that emulates a uniquely human trait: performing tasks based on verbal or written instructions and subsequently communicating them to others. This accomplishment addresses a…
NVIDIA’s GTC in Four Headlines
Impressive AI hardware innovations and interesting software moves….
It Is Possible to ‘Poison’ the Data to Compromise AI Chatbots With Little Effort – Technology Org
According to researchers, individuals could potentially disrupt the accuracy of AI chatbots by intentionally contaminating the datasets upon…